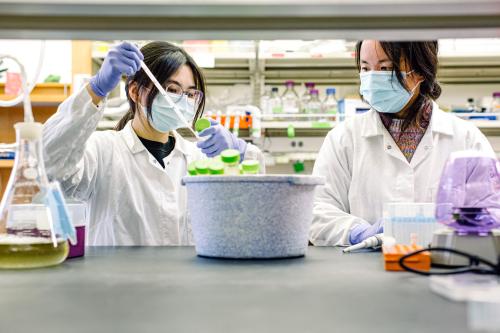
Tracing the steps of the ‘molecular dance’ between viruses and hosts
All living organisms are constantly evolving through random genetic mutations that arise as DNA replicates. Most of these mutations are neutral, but some can be helpful or harmful in the face of environmental challenges.
These challenges, called selective pressures, are key to evolutionary change. For hosts, selective pressures include deadly viruses, climate shifts or food scarcity. Viruses, on the other hand, face pressure from host immune systems, vaccines and antiviral drugs. When such pressures arise, individuals with mutations that confer an advantage are more likely to survive and pass their genes on to future generations.
The co-evolution of viruses and hosts – often likened to a molecular dance – has carried on across millions of years. As viruses evolve to better infect and replicate within hosts, the hosts develop new defensive measures. Viruses with mutations that enable them to either escape or antagonize these host defenses infect hosts yet again, driving a new cycle of evolutionary changes.
Thanks to advances in high-throughput sequencing and bioinformatics technologies, scientists can now gain an unprecedented view of the changes viruses and hosts have undergone to gain the upper hand.
Two new studies published in PLOS Pathogens and the EMBO Journal and led by Melody Li, Ph.D., of the UCLA Broad Stem Cell Research Center used these and other techniques to uncover intriguing new insights into this process. Here, Li breaks down the new findings and how they could be used to develop interventions to treat and curb the spread of dangerous viral infections.
Why study the evolution of viruses and hosts? How can it help us?
Viruses are constantly evolving, and we’re just racing to keep up. By studying the principles behind these evolutionary changes, both for specific viruses and broadly across many viral species, we can learn how viruses outsmart our immune systems and evade antiviral treatments.
Our ultimate goal is to predict how a virus is going to evolve, which would allow us to develop strategies to control, treat and maybe even eradicate it. On the host side, understanding how our antiviral defenses have evolved to fight viruses more effectively provides a sort of blueprint for creating better treatments.
How does your lab go about studying this evolution?
Every change in a viral or host protein leaves a molecular signature written into DNA, because DNA encodes proteins. We use an approach called evolutionary selection analysis to study DNA sequences and learn how genetic traits have changed over time as a result of natural selection.
Specifically, we look for sites in gene sequences where there have been more mutations that changed the function of proteins than mutations that did not. Those sites are likely to have been positively selected, meaning they held mutations that provided a survival advantage and thus were passed down to future generations.
To put it simply: Imagine 100,000 years ago, a deadly virus spreads through a population of early humans. Only those with a gene that produces a better antiviral protein will survive and pass on their genes to the next generation. Over time, after many such encounters with viruses, these beneficial genetic changes accumulate, leaving a record in our DNA sequences, much like fossils.
The study in PLOS Pathogens focuses on the human zinc finger antiviral protein (ZAP) – what’s special about this protein?
ZAP is crucial not only for human survival but for the survival of all species. It’s a really attractive protein to study because it’s broadly antiviral, meaning it can inhibit a wide array of viruses. This includes some of the deadliest and most intriguing viruses we’ve encountered, like Ebola virus and hepatitis B virus, as well as more common viruses like SARS-CoV-2.
What did the study uncover about ZAP?
As a first step, first author Serina Huang worked with evolutionary biologist David Enard, Ph.D., to create an alignment of ZAP sequences that generates a phylogenetic tree, which is like a family tree that shows evolutionary relationships between species. The tree she created revealed the genetic relatedness of the ZAP sequence across 261 mammalian species – from honey badgers to elephant seals to humans.
Serina then used evolutionary selection analysis to identify sites on the ZAP gene that showed signs of positive selection. Next, she generated mutant versions of these sites and tested their effects on alphavirus replication. One mutant, called N658A, was almost 10 times better at protecting against Sindbis virus than the version of ZAP found in humans.
The finding that our human version of ZAP isn’t the best it could be is really surprising. It also raises the question: Why don’t we have the best possible version of this extremely important protein?
How can this finding about ZAP be leveraged to advance treatments?
In addition to revealing that our version of ZAP could be better, the study uncovered new functions of specific parts of the protein that are critical to its ability to fight viruses. Fully understanding how all parts of ZAP work is the first step towards engineering broadly antiviral therapies that mimic this supercharged ZAP’s function to help patients fight infections.
This research could also be used to develop better attenuated vaccines. These vaccines prime our immune systems’ defenses by exposing us to viruses that have been weakened so they can’t cause disease. By understanding how our antiviral proteins target viruses, we can design more effective strategies to inactivate viruses for vaccines, potentially leading to a stronger immune response.
The study in the EMBO Journal focuses on chikungunya virus – what’s special about this virus?
Chikungunya virus is a mosquito-borne virus that was first identified in Tanzania in the 1950s. It started spreading more aggressively via a series of outbreaks in the early 2000s and now it has been seen in over 110 countries in Asia, Africa, Europe and the Americas.
The good thing is there’s a vaccine for chikungunya virus that was recently FDA approved. But kind of like what we’re seeing with long COVID, some people who’ve been infected with chikungunya virus experience long-term effects. A subset of patients has reported chronic arthritic pain for years after infection and a recent study of patient samples from an outbreak in Brazil found that the virus can also infect both the brain and spinal cord.
Basically, there are two concerning things about this virus: the fact that it’s evolved to become so good at spreading and the fact that it can cause these neurological consequences we’re just beginning to learn about.
What did the study uncover about chikungunya virus?
This paper, led by first author Zhenlan Yao, Ph.D., is focused on human immune cells called macrophages, which are found in nearly all tissues of the body and serve as a first line of defense against infection.
Many of the characteristics that make macrophages so great at fighting infection also make them prime targets for viruses that can hijack them to fuel their spread. Macrophages rush to the site of an infection, travel throughout the body and promote inflammation. Inflammation is good in small doses like when fighting an infection, but long-term, persistent inflammation causes all kinds of problems, like arthritis, which is inflammation of the joints.
Macrophages are known to be a viral reservoir for HIV, meaning that the virus can hide in them to evade immune detection and keep replicating, causing long-term infection. Zhenlan’s research confirms that macrophages can serve as a viral reservoir for chikungunya virus, which could explain both why the virus is spreading so effectively and why it can cause long-term arthritis.
How could this knowledge help us treat or stop the spread of chikungunya virus?
Knowing that chikungunya virus can hijack macrophages is crucial, but understanding how it does this is key to developing treatments. To investigate, Zhenlan analyzed over 300 viral genomic sequences from a global NCBI database using evolutionary selection analysis. She discovered that chikungunya viral glycoproteins disrupt cellular restriction factors within host cells, allowing the virus to produce more progeny.
This is exciting because it’s not what viral glycoproteins are known for. We usually think of them as sitting on the surfaces of viruses, where they bind to host cell receptors and facilitate viral entry. While we’ve long recognized their role in viral functions outside of host cells, this research shows that in the case of chikungunya virus, these glycoproteins also have a secret life inside host cells.
As a final step, Zhenlan used proteomics to identify two host antiviral proteins that directly interact with these viral glycoproteins, influencing the virus’s ability to produce more progeny in macrophages. Our lab is now pursuing further studies to understand how these interactions can be targeted therapeutically. If successful, such treatments could reduce the spread of the chikungunya virus and eliminate the viral reservoirs in the body.
Notes
The study published in PLOS Pathogens was funded by the National Institutes of Health, a UC Cancer Research Coordinating Committee Faculty Seed Grant, a UCLA AIDS Institute and Charity Treks 2019 Seed Grant and Johanna and Joseph H. Shaper Family Chair in Microbiology.
Additional authors are: Juliana Girdner, LeAnn P Nguyen, Carina Sandoval, Oliver I. Fregoso and David Enard.
The study published in the EMBO Journal was funded by the National Institutes of Health and a UC Cancer Research Coordinating Committee Faculty Seed Grant.
Additional authors are: Sangeetha Ramachandran, Serina Huang, Erin Kim, Yasaman Jami-Alahmadi, Prashant Kaushal, Mehdi Bouhaddou and James A. Wohlschlegel.